Hand-raising Orphan Asian Elephants
Chapter 5: Digestive Physiology & Gastrointestinal Flora
By Bhaskar Choudhary
With Contributions from Ellen Dierenfeld and Susan Mikota
Sections in this chapter include the following:
-
Introduction
-
Anatomy
-
Physiology
-
The microbiome
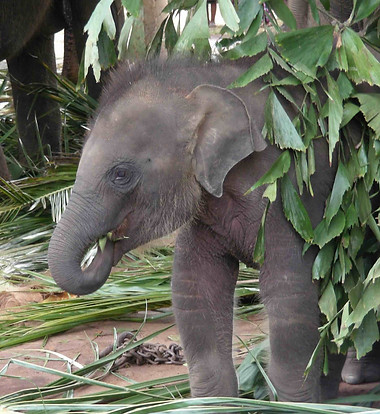
Photo credit: Susan Mikota
Introduction
Digestive disturbances are one of the most common causes of mortality in hand raised Asian elephant calves. Signs that may appear to be a simple gastrointestinal upset can rapidly progress to inappetence, diarrhea, coma, and acute death (Vijitha Perera and Bhaskar Choudhury Bhaskar, unpublished).
There can be many underlying causes, as discussed in Chapter 8 (Medical Problems and Treatment), however, the lack of a proven species-specific hand-raising formula for Asian elephants is a significant factor. While commercial formulas for Asian and African elephants have been developed (grobernutrition.com), these are expensive, not readily available in Asia, and we are not aware of any studies that show that they are superior to any of the human milk-based formulas that have been used.
The nursing success in Asian elephant calves is 35-40% at The Center for Wldlife Rehabilitation and Conservation (CWRC, Kaziranga Tiger Reserve, Choudhury, unpublished) which is largely attributed to formula limitations and associated gastrointestinal problems. Studies of Asian elephant milk have revealed that the composition varies throughout the long lactation (Abbondanza et al., 2013), presenting a challenge to developing a single milk replacer for elephants. The complexities of meeting the nutritional needs of the orphan calf are discussed in Chapter 4 (Feeding Milk).
Studies on the gut microbiota in recent years have been informative in further enhancing our understanding of the complex nature of digestion in Asian elephants, especially during the first few years of life (Ilmberger et al., 2014; Budd et al., 2020).
This chapter presents basic information about the anatomy and physiology of the Asian elephant gastrointestinal tract as well as recent studies about the elephant microbiome. As we learn more about the microbiome, we may be able to develop improved formulas that incorporate appropriate supplements to better support orphan Asian elephants in their early months of life.
Anatomy of the Gastrointestinal Tract
The elephant gastrointestinal (GI) tract includes the mouth, pharynx, esophagus, a simple stomach, small and large intestines, and the anus. Additional organs, such as molar teeth, tongue, salivary glands, liver and pancreas, complete the GI system. Elephants do not have a gall bladder.
The elephant oral cavity is relatively small compared to the body size. The tongue is attached to the floor of the mouth which prevents it from protruding, but it can fold in the center which helps to move food to the back of the throat. Some but not all calves are born with teeth (See Table 2.3). Over the course of its lifetime an elephant will have a total 24 molar teeth (six on each upper side and six on each lower side). As teeth wear down, they are shed in sections and are replaced by the next tooth which emerges horizontally (unlike in most mammals in which teeth emerge vertically). Table 8.4 shows the age at which molars erupt and are replaced. Teeth are discussed further in Chapter 2 (Physical Examination and Preventive Healthcare Practices) and Chapter 8 (Medical Problems and Treatment).
The elephant has a simple stomach. The duodenum, jejunum, and ileum comprise the small intestines; the cecum, colon, rectum, and anal canal comprise the large intestines. Figure 5.1 illustrates Asian and African GI tracts. Compared to other herbivorous species, both elephant species have a short intestinal tract; Asian elephants have longer large and small intestines than African elephants. The volume of the various sections of the GI tract have been measured in adult elephants but not in neonates.
Physiology of the Gastrointestinal Tract
Elephants are monogastric (one stomach), herbivorous, non-ruminant, hindgut fermenters. Hindgut fermenters ingest and process food more rapidly than foregut fermenters and this may have facilitated the evolution of their large body size. The cecum is the first important fermentation site, but the colon is the main digestive area.
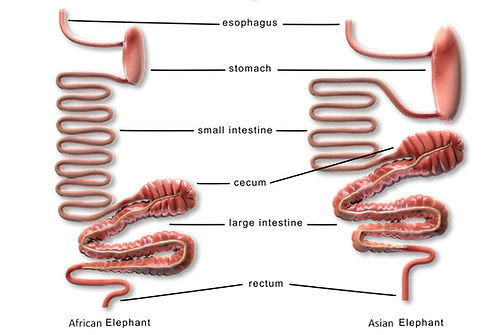
Figure 5.1 Comparison of African and Asian elephant GI tracts (Greene et al., 2019)
Elephants are generalized feeders and consume large amounts of low-quality forage that pass through their gut in a relatively short period of time (Loehlein et al., 2001). Asian elephants digest only ~ 40–50% of the forage that they consume (Sukumar, 2006).
The natural diet of the adult Asian elephant typically includes a high proportion of grasses but is habitat dependent (Dierenfeld, 2006). Adult Asian elephants are more likely to consume browse during the dry season, while both Asian and African elephants browse and consume grasses during the rainy season (Koirala et al., 2016). Elephants favor leaves and twigs more than other plant parts and there is a negative correlation between plant availability and preference. So, food selection is not dictated by relative availability – rather elephants may have specific individual preferences (Koirala et al., 2016).
Gastrointestinal motility depends on a complex interaction of neural, hormonal, vascular and neuromuscular pathways as well as the type of food eaten. A diet of concentrates and hay will have a faster transit time than a diet of only hay.
GI transit time is basically the time for food to move from mouth to anus and is between 21-55 h in adult Asian elephants. African elephants have a faster transit time than Asian elephants. Some GI studies calculate mean retention time which is the mean # of hours from 1st to last appearance of an orally administered marker. In an early study, adult elephants were fed ~ 200 1-cm diameter O-rings; defecations were recorded and washed through a sieve to determine times at which the rings they were excreted. Orange peels have also been used to determine GI transit time, as they are not digested and will appear intact in the feces. Although elephants lack a gall bladder, bile is continuously secreted into the small intestine through multiple ducts.
Adult elephants defecate 15-20 times daily, passing 5-8 fecal balls (boli) each time. A fecal bolus can weigh 1 – 2.5 kg (Cheeran, 2002).
Introduction to the Microbiome
The microbiome is defined as the community of microorganisms (including bacteria, fungi, viruses and their genomes) that inhabit a particular environment. In animals, the term describes the microorganisms that live in or on a particular part of the body, such as the skin or gastrointestinal tract.
The importance of the microbiome in humans and animals to overall health, welfare, and even behavior, has only recently been recognized. We know the microbiome is critical to healthy digestive function and immunity but increasing evidence links the microbiome to many other functions. See Figure 5.2.
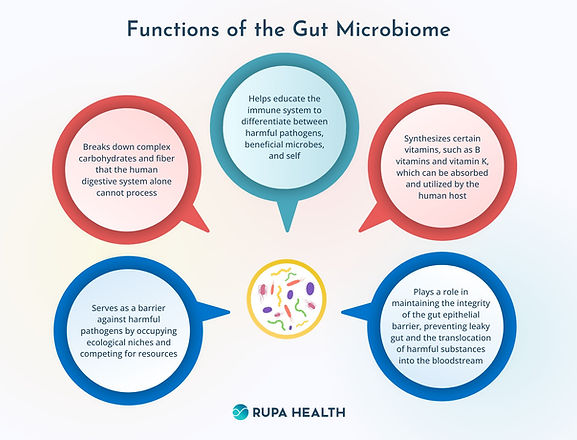
Figure 5.2 Functions of the Gut Microbiome. Source: https://www.rupahealth.com/post/what-is-the-gut-microbiome
The most comprehensive and integrative summary of factors impacting elephant gut microbiome published to date examined relationships among metabolic and reproductive hormones, clinical health issues, and fecal microbiota in 69 African and 48 Asian female elephants from 50 US zoos (Keady et al., 2021). In this study, both species showed similar primary and dominant phyla – likely a result of limited managed dietary ingredients— but distinct species differences (genetic component) were identified in both richness and bacterial composition, with Asian elephants higher in both indices compared to Africans.
Zoo location (environment) impacted microbial populations, as did some clinical health issues. Species-specific associations between gut microbiomes and hormones were found: Asian elephant gut microbiome composition was linked to thyroid hormone metabolites, whereas African elephant gut microbes were correlated with changes in fecal glucocorticoid metabolites (possibly stress indicators).
Various relationships were identified between specific microbial relative abundances and health concerns (obesity, lameness) as well as between microbial species and hormone concentrations, with the authors concluding that Prevotella, Treponema, and Akkermansia species in particular might be useful targets for more intensive study regarding health and reproduction in elephants.
Studies of elephant gut microbiome descriptions (Ilmberger et al., 2014; Zhang et al., 2019; Budd et al., 2020; Kambe et al., 2020; Keady et al., 2021; Moustafa et al., 2021) - determined through fecal sampling - all demonstrate a highly diverse microbial population in older animals dominated primarily and unsurprisingly (as in other herbivores) by species typically within the phyla Firmicutes, Bacteroidetes, often Proteobacteria, and to a lesser extent, Spirochaetes or Verrucomicrobia (the latter in managed care only). Captive individuals of both African and Asian elephants compared with free-ranging animals display differences in gut microbial populations that appear to reflect not only diets and environments but also, at a finer scale, species-specific impacts, with further detailed relationships yet to be explored.
Recent work conducted with Asian elephants has revealed a highly diverse flora including Firmicutes, Bacteroides, Spirochetes, Bifidobacteria, and Proteobacteria species predominating. In one study, fecal microbiomes of semi-free-range Asian elephants in Myanmar differed seasonally, with Firmicutes and Bacteroides species dominant in the dry season and more Proteobacteria and Spirochetes present in the rainy season, indicating an influence of diet on microbes. This same study showed that Albendazole temporarily impacted the gut microbiome, but the microflora returned to pre-treatment levels within three weeks (Moustafa et al., 2021).
Detailed recent publications (Klinhom et al., 2023; Klinsawat et al., 2023) on the influence of age (and associated diet – both ingredients and nutritional quality, also linked with geography) on microbiome through fecal characterization in Thai Asian elephants revealed distinct differences across groups.
Firmicutes, Bacteroidetes, Spirochaetota and Actinobacteria were identified as dominant phyla across all age groups. However, milk-drinking infants and suckling calves showed the lowest diversity (23 phyla, ~400 genera); milk-fermenting taxa Bifiodobacterium and Akkernansia species dominated in infants, whereas more Lachnospiraceae species (fiber-fermenting taxa) were identified in older suckling calves. Added microbial populations are likely inoculated through natural exploratory behaviors, including testing new plant-based foodstuffs and coprophagy (Figure 5.3) as animals mature.
Weaned calves (3-year), subadults, and adults (>10-14 years) demonstrated higher microbial diversity (33 phyla, ~600 genera identified – again, predominantly fiber-digesting species that remained relatively stable through these age classes. Geriatric elephants in this study (Klinhom et al., 2023) displayed distinctly different microbial populations from other age groups, with a decline in microbial diversity that may be associated with tooth loss, different dietary preferences and/or digestive processes.

Figure 5.3 Coprophagy may help to establish the GI micobiome. Photo credit: Bhaskar Choudhury
Both Thai studies demonstrated high diversity within the gut microbial populations in managed Asian elephants, with many similarities to predominant microbiomes described for both semi-wild and managed Asian elephants elsewhere, as well as African elephants (Klinsawat et al., 2023). However, some unique microbes were identified by Klinsawat et al. (2023), and particular differences in microbiomes in elephants fed predominantly local palms (Caryota urens) compared with Napier grass (Pennisetum purporeum)-based diets may have broader health and nutrition implications for the species.
Thus, age of the animal can play a huge role in gut microbiome diversity, with diet likely playing the predominant role. Both Ilmberger et al. (2014) and Kambe et al. (2020) demonstrated that elephant calves have a much less diverse microbiome compared with older individuals, but changes can develop rapidly in the very young calf.
In an elephant study (with a single individual), Kambe et al. (2020) documented radical differences in microbial diversity, community composition, and specific taxa (order) between mother and calf. Enterobacteroidales and Lactobacillales were initially found in the infant; since lactic acid bacteria stimulate gut immunity and barrier in other species, these may be critically important components of the early elephant calf gut development. Milk substrates positively correlated with those particular microbial constituents include the sugars lactose, rhamnose, sorbitol, fucose, and sorbose, along with protein constituents asparagine and threonine, as well as the hormone estradiol-17ß (Lactobacillales only).
These sugar components in milk appeared in highest quantities up to about Day 7 of lactation; later (Days 22-37) milk samples analyzed higher in specific amino acid (aspartic acid) and lipid (palmitic acid) components. Concurrent with these changes in milk constituents, by Day 6, Bacteroidales predominated and by Day 29, Clostridiales was identified as the primary bacterial order in the calf, which also positively correlated with milk palmitic acid content.
By one month of a milk-based diet, the calf microbiome more closely resembled phyla proportions found in the mother. Interestingly, this calf was observed practicing coprophagy at Day 26, which may also have contributed to the changeover in gut microbes. In other species, oligosaccharides are involved in the increase of lactic acid bacteria in the gut environment; oligosaccharides and glucosamine have been identified in Asian elephant milks (Uemura et al., 2006; Takatsu et al., 2017) and may also play a role in elephant calf gut microbiome development.
Detailed analyses that correlate diet chemistry and fecal bacteria may help us better understand the relationship(s) of dietary constituents in milk to optimize the gut microbiome, development, and associated health of elephant calves, beginning at birth.
In the earliest published elephant microbiome report comparing the gut microbiome in a three-week-old (milk-fed) calf versus a six-year-old elephant, Ilmberger et al. (2014) also documented Bacteroidetes and Firmicutes as predominant phyla. Proteobacteria species, however, were found in a high proportion in the calf feces of their study – likely due to dietary substrate differences.
These authors found many unique microbial carbohydrase enzymes in both samples analyzed, and suggested that Bacteroidetes species seemed to play a critical role in carbohydrate utilization in elephants, perhaps warranting focus in future targeted probiotic blends for elephants. Unpublished data (Paine and Choudhury) demonstrated significant differences in microbiome between a newborn calf and its mother, which the authors attributed to microbial exposure in the birth canal, as well as milk, resulting in an early dominance of Firmicutes in the calf.
A later microbiome study of Zhang et al. (2019) with three free-range elephants in China reported that Firmicutes species were found in greater abundance than Bacteroidetes species, and a number of unique carbohydrases were detected in fecal samples. Fibrobacteres species also detected in this study increased proportionately with age (and presumably dietary fiber), but overall microbiome diversity decreased.
These authors found evidence for greater hemicellulose- than cellulose-degrading enzymes in the microbial metagenomic evaluation of samples, and suggested hemicellulose as the primary energy source for elephants from this locale. Diets consumed by elephants in this study comprised bamboo and plantain; it’s possible that the microbes and metagenomics of this particular population may reflect that unique landscape/habitat and diet rather than a broader elephant microbiome generalization. Bamboo, as a grass, typically contains a higher hemicellulose component than other plant types, thus microbial adaptation to hemicellulose (a substrate which can also be partially hydrolyzed by stomach acids prior to microbial exposure in the hindgut) appears to be a useful adaptive digestive strategy.
In adult elephants, environment and accompanying diet have been shown to influence microbial species abundance as well as, in some cases, diversity. African savannah elephants (Loxodonta africana) display a majority Firmicutes species typically associated with grazing hindgut fermenters, whereas the microbiome of African forest elephants (L. cyclotis) was dominated by Proteobacteria, similar to more frugivorous species (Budd et al. 2020).
Specific community composition, but not microbial diversity, was seen to change in free-range compared to captive Asian elephants; diversity increased in one study with animals stressed by translocation, with an increase in Bacteroidetes species and decrease in Proteobacteria seen in response to both anthropogenic activities described. Additionally, as mentioned above, effects of management activities (a specific deworming agent, Albendazole) temporarily impacted the gut microbiome, decreasing Firmicutes and Spirochaetes while increasing Proteobacteria proportions; direct and indirect effects of the medication were documented but the microflora resorted to pre-treatment levels within 3 weeks (Moustafa et al., 2021).
In an unpublished study, Paine and Choudhury reported the wild Asian elephant gut microbiome collected from animals (n=9) in grasslands of the Brahmaputra Valley was dominated by Firmicutes (23%-47%) and Bacteroidetes (20-60%) species, and differed significantly from captive adults which displayed Thermoplasmata (~40%), Cyanobacteria (~45%) and Methanobacteria (~10%). The wild elephants also displayed a unique range of soil microbes in fecal samples, that may be associated with plants (and/or other substrates) consumed in natural environments. Imbalances in the microbiome may be involved in some of the GI-related medical problems that we see in adult as well as neonatal elephants.
As we begin to better understand more about the elephant microbiome, we may be able to use this knowledge therapeutically to optimize health and nutrition. While a healthy gut microbiome is critical to digestive function and immunity, increasing evidence links specific microbial interaction to endocrine control of metabolic and reproductive hormones which, in turn regulate physiological responses, metabolism, mood, growth and development, and reproduction.
We need to acknowledge species-specific genetics, environmental and dietary factors that impact the gut microbiome, and better understand relationships among microflora, endocrine hormones and overall health in situational management of gut dysbiosis. Such issues are not trivial and can have lifelong impacts regarding health of the individual, and provide relevant information for niche identification, rehabilitation and conservation of the species.
From a practical starting perspective, faunation or transfaunation can occur naturally through exposure during birth, suckling, normal dietary consumption, and/or coprophagy. In response to dysbiosis, animals can be orally transplanted with gut microbiota from other healthy individuals through fecal harvest following discontinuation of antimicrobials (Mullen et al., 2018) with or without freezer storage for future use in animal treatment/management. Feeding inulin (a prebiotic) may also help to restore the gut microbiome in elephants with colic (Bo et al., 2023).
Literature Cited
Abbondanza, F.N., Power, M.L., Dickson, M.A., Brown, J., Oftedal, O.T., 2013. Variation in the Composition of Milk of Asian Elephants (Elephas maximus) Throughout Lactation. Zoo Biology 32, 291-298.
Bo, T., Liu, H., Liu, M., Liu, Q., Li, Q., Cong, Y., Luo, Y., Wang, Y., Yu, B., Pu, T., Wang, L., Wang, Z., Wang, D., 2023. Mechanism of inulin in colic and gut microbiota of captive Asian elephant. Microbiome 11, 148.
Budd, K., Gunn, J.C., Finch, T., Klymus, K., Sitati, N., Eggert, L.S., 2020. Effects of diet, habitat, and phylogeny on the fecal microbiome of wild African savanna (Loxodonta africana) and forest elephants (L. cyclotis). Ecology and evolution 10, 5637-5650.
Cheeran, J.V., 2002. Elephant facts. Journal of Indian Veterinary Association Kerala 7, 12-14.
Dierenfeld, E., 2006. Nutrition. In: Fowler, M.E., Mikota, S.K. (Eds.), Biology, Medicine, and Surgery of Elephants . Blackwell Publishing Ltd.
Greene, W., Dierenfeld, E.S., Mikota, S., 2019. A review of Asian and African elephant gastrointestinal anatomy, physiology and pharmacology. Journal of Zoo and Aquarium Research 7, 1-14.
Ilmberger, N., Guellert, S., Dannenberg, J., Rabausch, U., Torres, J., Wemheuer, B., Alawi, M., Poehlein, A., Chow, J., Turaev, D., Rattei, T., Schmeisser, C., Salomon, J., Olsen, P.B., Daniel, R., Grundhoff, A., Borchert, M.S., Streit, W.R., 2014. A Comparative Metagenome Survey of the Fecal Microbiota of a Breast- and a Plant-Fed Asian Elephant Reveals an Unexpectedly High Diversity of Glycoside Hydrolase Family Enzymes. PLoS ONE 9, e106707.
Kambe, J., Sasaki, Y., Inoue, R., Tomonaga, S., Kinjo, T., Watanabe, G., Jin, W., Nagaoka, K., 2020. Analysis of infant microbiota composition and the relationship with breast milk components in the Asian elephant (Elephas maximus) at the zoo. The Journal of veterinary medical science 82, 983-989.
Keady, M.M., Prado, N., Lim, H.C., Brown, J., Paris, S., Muletz-Wolz, C.R., 2021. Clinical health issues, reproductive hormones, and metabolic hormones associated with gut microbiome structure in African and Asian elephants. Anim Microbiome 3, 85.
Klinhom, S., Sriwichaiin, S., Kerdphoo, S., Khonmee, J., Chattipakorn, N., Chattipakorn, S.C., Thitaram, C., 2023. Characteristics of gut microbiota in captive Asian elephants (Elephas maximus) from infant to elderly. Sci Rep 13, 23027.
Klinsawat, W., Uthaipaisanwong, P., Jenjaroenpun, P., Sripiboon, S., Wongsurawat, T., Kusonmano, K., 2023. Microbiome variations among age classes and diets of captive Asian elephants (Elephas maximus) in Thailand using full-length 16S rRNA nanopore sequencing. Sci Rep 13, 17685.
Koirala, R.K., Raubenheimer, D., Aryal, A., Pathak, M.L., Ji, W., 2016. Feeding preferences of the Asian elephant (Elephas maximus) in Nepal. BMC Ecol 16, 54.
Loehlein, W., Kienzle, E., Wiesner, H., Clauss, M., 2001. Investigations on the Use of Chromium Oxide as an Inert, External Marker in Captive Asian Elephants (Elephas maximus): Passage and Recovery Rates. In, A Research Update on Elephants and Rhinos; Proceedings of the International Elephant and Rhino Research Symposium, Vienna, June 7-11, 2001, 250-250.
Moustafa, M.A.M., Chel, H.M., Thu, M.J., Bawm, S., Htun, L.L., Win, M.M., Oo, Z.M., Ohsawa, N., Lahdenperä, M., Mohamed, W.M.A., Ito, K., Nonaka, N., Nakao, R., Katakura, K., 2021. Anthropogenic interferences lead to gut microbiome dysbiosis in Asian elephants and may alter adaptation processes to surrounding environments. Sci Rep 11, 741.
Mullen, K.R., Yasuda, K., Divers, T.J., Weese, J.S., 2018. Equine faecal microbiota transplant: Current knowledge, proposed guidelines and future directions. Equine Veterinary Education 30, 151-160.
Paine and Choudhury (2018), unpublished.
Sukumar, R., 2006. A brief review of the status, distribution and biology of wild Asian elephants. . International Zoo Yearbook 40, 1-8.
Takatsu, Z., Tsuda, M., Yamada, A., Matsumoto, H., Takai, A., Takeda, Y., Takase, M., 2017. Elephant's breast milk contains large amounts of glucosamine. J Vet Med Sci 79, 524-533.
Uemura, Y., Asakuma, S., Yon, L., Saito, T., Fukuda, K., Arai, I., Urashima, T., 2006. Structural determination of the oligosaccharides in the milk of an Asian elephant (Elephas maximus)412. Comp Biochem. Physiol A Mol. Integr. Physiol 145, 468-478.
Zhang, C., Xu, B., Lu, T., Huang, Z., 2019. Metagenomic Analysis of the Fecal Microbiomes of Wild Asian Elephants Reveals Microflora and Enzymes that Mainly Digest Hemicellulose. Journal of microbiology and biotechnology 29, 1255-1265.